Nigel Syrotuck, Mechanical Engineer, StarFish Medical10.17.18
More often than you might expect, magnetism plays a key role in the medical device commercialization process. Permanent magnets are used to affix components together for ingress protection and to improve cleanability—the magnets can be hidden behind sealed plastic or non-magnetic metal walls without exposing a seam or grooves that collect dirt or biological debris. Magnetic switches can also be used as interlocks or for emergency shutoffs to ensure safety. Sometimes, magnets are behind the core technology of a device and the commercialization team has to accommodate, enhance, or avoid interference with the magnetic field generated by other device features.
In this article, practical magnetics from a mechanical engineering perspective will be discussed as it pertains to medical devices. (Electronics, including the design of electromagnetics, EMI, soft versus hard magnetism, and induction will be saved for another day.)
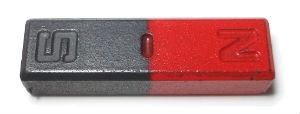
A bar magnet.
What Is Magnetism?
Magnetism is a class of physical phenomena characterized by force fields. Magnetism cannot be defined by classical physics and is regarded as a quantum effect (it’s not just you that doesn’t really understand magnets!) That said, we do know magnetic fields are caused by electron spin and exchange integration, which create magnetic fields mostly in metals. These effects result in micro-domains that create local magnetic fields; however, ferromagnetic materials aren’t normally outwardly magnetized because the micro-domains typically point in different, random directions, canceling each other out. When a strong magnetic field is presented (such as by a permanent magnet), the fields align temporarily and the materials attract. The degree to which materials do this is called magnetic permeability. If the magnetic field is extremely strong, the domain walls will actually re-orient and the material becomes a permanent magnet.
This is an example of micro-domains.
Permanent Magnets
The most common type of permanent magnet is a neodymium magnet, which is made up of neodymium, iron, and boron. They are available in various grades, with N52 being the strongest and most expensive. They will lose their magnetism above their Curie temperature, but that temperature is typically above 300oC. They are vulnerable to corrosion, so most commercial versions are coated in a layer of nickel to protect them. However, this layer tends to be brittle and chip off, therefore alternate coatings are more appropriate for corrosive environments.
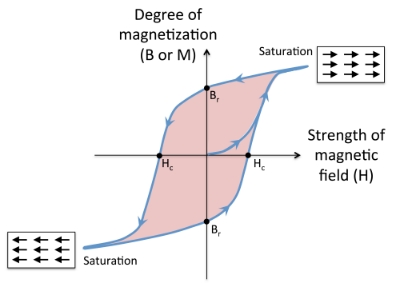
Hysteresis loop for a ferromagnet.
Hysteresis
Even if not turned into a permanent magnet, ferromagnetic materials have a tendency to stay magnetized to some extent after being subjected to an external magnetic field. This phenomenon is called hysteresis. The degree to which hysteresis is present is called the remanence of the material, and though it is an important factor in the design of electromagnets and permanent magnets, it tends to be an insignificant effect in medical devices—except where magnetics are used as sensors.
All About Metals
(Note that ferromagnetism is described here, as opposed to the other types of magnetism (para, dia, and anti-ferromagnetism), which tend to be both weaker (i.e. less practical) and more complex.)
Alloys with iron (e.g. steel), cobalt, and nickel are generally magnetic. These are commonly found in general structural parts, implants, and instruments respectively. Some alloys common in medical devices, such as certain types of stainless steels, chrome-cobalt implants, and nitinol (shape memory alloy wire) contain these elements but aren’t magnetic due to their resultant crystalline structure. The chart below shows very common medical device metals and their magnetism, though it’s highly dependent on the manufacturing, treatment, and handling processes used to produce a part.
* when annealed to increase magnetic properties
**unless annealed to remove magnetic effects
***…as a general rule. Actual magnetism is relative and highly dependent on processing (such as working, welding, or annealing). In units of μ/μ0 (relative permeability) where vacuum is defined as exactly 1.
Modeling Magnetic Fields
Ferromagnetic metals that don’t emanate a unified magnetic field (e.g., a “non-magnetized” piece of iron) interact with magnetic fields emanating from permanent or electromagnets in a simplified way. They act as a conductor of magnetic fields, allowing the magnetic fields to take the path of least resistance, much like electricity prefers metallic conductors to air. In magnetics, this not only causes their magnetic domains to align but also affects the magnetic field map. If the magnetic field lines are small streams, ferromagnetic materials are the river that absorbs all streams that try to cross it. Similarly, the "streams" affect the “river” itself—if the streams are small (the nearby magnet is weak), then the river will be largely unchanged (little magnetic alignment). If the streams are large (the nearby magnet is strong), then the river will swell (become a magnet itself). Again, this is also true for electricity, which will travel along conductors to conserve energy by following the path of least resistance. The practical application of all this is that magnetic fields can be altered and shaped in the desired way by understanding the path of least resistance and using ferromagnetic materials in the vicinity of the magnetic field source. Modeling software is the simplest way to visualize this effect and show the resultant fields.
In this article, practical magnetics from a mechanical engineering perspective will be discussed as it pertains to medical devices. (Electronics, including the design of electromagnetics, EMI, soft versus hard magnetism, and induction will be saved for another day.)
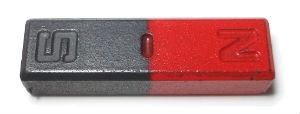
A bar magnet.
Magnetism is a class of physical phenomena characterized by force fields. Magnetism cannot be defined by classical physics and is regarded as a quantum effect (it’s not just you that doesn’t really understand magnets!) That said, we do know magnetic fields are caused by electron spin and exchange integration, which create magnetic fields mostly in metals. These effects result in micro-domains that create local magnetic fields; however, ferromagnetic materials aren’t normally outwardly magnetized because the micro-domains typically point in different, random directions, canceling each other out. When a strong magnetic field is presented (such as by a permanent magnet), the fields align temporarily and the materials attract. The degree to which materials do this is called magnetic permeability. If the magnetic field is extremely strong, the domain walls will actually re-orient and the material becomes a permanent magnet.
This is an example of micro-domains.
Permanent Magnets
The most common type of permanent magnet is a neodymium magnet, which is made up of neodymium, iron, and boron. They are available in various grades, with N52 being the strongest and most expensive. They will lose their magnetism above their Curie temperature, but that temperature is typically above 300oC. They are vulnerable to corrosion, so most commercial versions are coated in a layer of nickel to protect them. However, this layer tends to be brittle and chip off, therefore alternate coatings are more appropriate for corrosive environments.
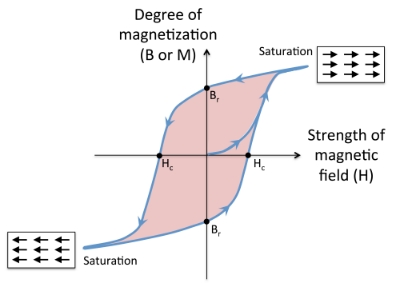
Hysteresis loop for a ferromagnet.
Even if not turned into a permanent magnet, ferromagnetic materials have a tendency to stay magnetized to some extent after being subjected to an external magnetic field. This phenomenon is called hysteresis. The degree to which hysteresis is present is called the remanence of the material, and though it is an important factor in the design of electromagnets and permanent magnets, it tends to be an insignificant effect in medical devices—except where magnetics are used as sensors.
All About Metals
(Note that ferromagnetism is described here, as opposed to the other types of magnetism (para, dia, and anti-ferromagnetism), which tend to be both weaker (i.e. less practical) and more complex.)
Alloys with iron (e.g. steel), cobalt, and nickel are generally magnetic. These are commonly found in general structural parts, implants, and instruments respectively. Some alloys common in medical devices, such as certain types of stainless steels, chrome-cobalt implants, and nitinol (shape memory alloy wire) contain these elements but aren’t magnetic due to their resultant crystalline structure. The chart below shows very common medical device metals and their magnetism, though it’s highly dependent on the manufacturing, treatment, and handling processes used to produce a part.
Common Name: | 200 Series Stainless Steel | 300 Series Stainless Steel (austenitic) | 400 Series Stainless Steel | Duplex Steel | 17-4PH | Stainless Steel Welds, casts | Plain Carbon Steel | Cobalt Chrome | Nitinol | ||||
Includes: | 201, 202, 205, etc. |
301,302, 303, 304, 321, 347 18/8, A2 |
316, Sometimes 18/8 |
310, 305 | Ferritic: 430 and 446 |
Martensitic |
2XXX series |
630, 1.4542 (EU) |
N/A | Numerous Grades | Nitinol 55 and Nitinol 60 | ||
410, 420, 440 |
416 | ||||||||||||
Corrosion Resistance: | Fair | Good | Excellent | Excellent | Good | Good | Fair | Good | Good | Various | Poor, unless coated | Excellent | Good |
Main Characteristics: | Cheap, Hard, Strong |
Biocompatible, autoclavable, weldable 310 is more stable at high temperatures but more expensive than 400 series |
Wear resistant | Wear resistant | Machinable | Strong, harder to machine | Cheaper, lighter, high strength | Different alloys | Cheap and strong | Inert, hard, biocompatible | Bendable, reverts shape | ||
Relative Magnetism: *** |
Slightly magnetic (1.004-3**) |
Slightly magnetic1 (1.004-3**) |
Non-magnetic2 (~1.003-1.01) Especially LN types |
Non-magnetic1 (~1) |
Magnetic2 1000-1800* |
Magnetic2 750-950* |
Magnetic: 750* |
Magnetic, (halfway between austenitic and ferritic alloys) |
Magnetic (similar to martensitic alloys) |
Can be fairly magnetic3 |
Magnetic ~1004 |
Non-magnetic, Typically safe for MRI |
Non-magnetic (<1.002)5 |
**unless annealed to remove magnetic effects
***…as a general rule. Actual magnetism is relative and highly dependent on processing (such as working, welding, or annealing). In units of μ/μ0 (relative permeability) where vacuum is defined as exactly 1.
Modeling Magnetic Fields
Ferromagnetic metals that don’t emanate a unified magnetic field (e.g., a “non-magnetized” piece of iron) interact with magnetic fields emanating from permanent or electromagnets in a simplified way. They act as a conductor of magnetic fields, allowing the magnetic fields to take the path of least resistance, much like electricity prefers metallic conductors to air. In magnetics, this not only causes their magnetic domains to align but also affects the magnetic field map. If the magnetic field lines are small streams, ferromagnetic materials are the river that absorbs all streams that try to cross it. Similarly, the "streams" affect the “river” itself—if the streams are small (the nearby magnet is weak), then the river will be largely unchanged (little magnetic alignment). If the streams are large (the nearby magnet is strong), then the river will swell (become a magnet itself). Again, this is also true for electricity, which will travel along conductors to conserve energy by following the path of least resistance. The practical application of all this is that magnetic fields can be altered and shaped in the desired way by understanding the path of least resistance and using ferromagnetic materials in the vicinity of the magnetic field source. Modeling software is the simplest way to visualize this effect and show the resultant fields.
5 http://citeseerx.ist.psu.edu/viewdoc/download?doi=10.1.1.467.425&rep=rep1&type=pdf
Nigel Syrotuck is a mechanical engineer at StarFish Medical. His background includes a diverse project development portfolio including sustainable power solutions, assisted living devices, and nano-satellite design.
Nigel Syrotuck is a mechanical engineer at StarFish Medical. His background includes a diverse project development portfolio including sustainable power solutions, assisted living devices, and nano-satellite design.